In 1969, amidst the Cold War, the crew of a Soviet ship found themselves grappling with boredom. Seeking to alleviate their monotony, they decided to write a letter, place it in a bottle, and cast it into the vast ocean. Despite the tense relationship between the Soviet Union and the United States at that time, the message contained no threats, but rather conveyed warm greetings and innocent wishes. Fast forward fifty years, and fate intervened when someone stumbled upon this very bottle on the shores of Alaska. Through this unexpected discovery, we were presented with tangible evidence of the ship’s existence and its sailor, Anatoliy Botsanenko, who penned the letter. In reflecting on the motive behind their seemingly absurd act, Botsanenko explained, “During one monotonous evening, my friends pondered a question: Will there come a time when we are forgotten, and the existence of this ship and its crew fades into obscurity?”
While Anatoliy and his comrades cast their message into the ocean without any expectation of it being found, a different narrative unfolded during that era. A group of scientists eagerly awaited a message from the vast expanse of the universe. Armed with nothing but scientific certainty, they believed that one day, with remarkable fortune and tremendous dedication, they would capture this elusive message. The arrival of this long-awaited communication on September 14, 2015, was a cause for immense jubilation for all of humanity. It was intercepted by one of the twentieth century’s most remarkable accomplishments, known as “LIGO.”
This message was titled: “Gravity Waves.”
A Violin Player and his Strings
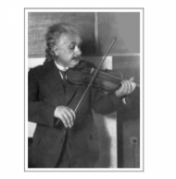
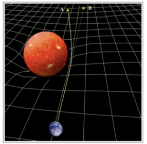
The bending of light due to the gravitational influence, as described by Albert Einstein’s theory of general relativity.
In order to grasp the concept of gravity, we must embrace the notion that it is no longer an invisible force pulling objects together based on mass, acceleration, and distance, as Isaac Newton proposed. Newton’s contributions in explaining and interpreting the effects of gravity on our daily lives are undoubtedly commendable, as he formulated elegant and straightforward laws. However, as the inheritors of the post-relativity era, we have come to recognize that gravity is, in fact, the result of the curvature or alteration of the fabric of space-time. The intertwined entities of space and time collectively constitute the fabric of the universe, and they are influenced by the presence of mass. This pristine fabric becomes contorted, giving rise to bends and distortions, which we understand as gravity.
It appears that the initial mention of gravitational waves can be traced back to an article penned by Henri Poincaré in 1905. In this article, Poincaré provided a summary of his mathematical theory of relativity and introduced the concept of a “gravitational wave” as the means through which gravity is transmitted. Subsequently, in 1915, Einstein, after publishing his theory of general relativity, predicted the existence of gravitational waves as a consequence of the movement of matter or the collision of massive objects within the fabric of space-time. Remarkably, this prediction was eventually confirmed a century later when gravitational waves reached us. The most visually intuitive demonstrations of gravitational waves may lead us to believe that they are a logical outcome of the general theory of relativity’s principle of gravity. However, these waves proved to be a significant challenge for Einstein, who treated them similarly to electromagnetic waves. In the case of electromagnetic waves, radiation arises from the vibrations of an electric dipole, which consists of two charges—one positive and the other negative. The oscillations of such an electric dipole within a given space generate an electromagnetic wave. Conversely, in the realm of gravity, the analogy falls apart because negative mass does not exist. This absence of negative mass ultimately prompted Einstein to abandon the concept of gravitational waves.
Nonetheless, Einstein remained unconvinced about the nonexistence of these waves. He persisted in grappling with intricate mathematics, seeking solutions akin to the efforts of the scientist Maxwell in the realm of electrodynamic wave equations. Consequently, Einstein proposed three different types of gravitational waves, albeit without complete satisfaction. These proposals were left to linger for a considerable period, awaiting the attention of a scientist of Einstein’s stature, specializing in theoretical physics and the founding father of general relativity. Perhaps Einstein’s preoccupation with gravitational waves stemmed from the emergence of a groundbreaking theory that rocked the physics community: quantum mechanics. Additionally, his fascination with the cosmic constant, which he introduced to maintain a static universe, was another factor. Some physicists had presented a logical analysis indicating that general relativity actually predicted an expanding universe. Consequently, the question of gravitational waves continued to haunt prominent figures within the realm of physics. In 1922, Sir Arthur Eddington, the physicist and astronomer who verified general relativity during the 1919 eclipse, published a scientific paper shedding light on the matter. Eddington explained that the two types of gravitational waves identified by Einstein traveled at different speeds within a “wavy” coordinate system in flat spaces, and they were not waves in the fabric of space-time. In his calculations, he focused on the third wave and demonstrated that it travels at the speed of light in all directions. Therefore, the existence of gravitational waves remained a possibility and was not ruled out.
Twenty years following the publication of the theory of general relativity, Einstein corresponded with his esteemed physicist friend, Max Born, stating, “My young assistant Rosen and I have reached an intriguing conclusion: gravitational waves do not exist.” Subsequently, on June 1, 1936, Einstein and Rosen submitted an article titled “Are there gravitational waves?” to the esteemed journal Physical Review, which was then under the editorship of John Tate. Tate, in turn, presented the article to Howard Robertson for careful evaluation. Robertson provided critical feedback, expressing reservations about the manuscript. On July 23, John Tate forwarded the reviewer’s comments to Einstein, without disclosing the reviewer’s identity. This course of action displeased Einstein, leading him to respond indignantly in his letter:
“Dear Sir,
Mr. Rosen and I submitted our manuscript for publication, with the understanding that it would not be shared with specialists prior to its printing. Consequently, I fail to discern any justification for reviewing the ‘negative comments’ from your anonymous expert. In light of these circumstances, I would prefer to seek publication elsewhere.
Einstein”
Einstein found it frustrating that his paper had been reviewed without his permission. However, an interesting development occurred when Robertson, “the peer-reviewer of Einstein’s paper,” formed a friendship with Leopold Infeld, a young physicist who replaced Rosen as Einstein’s new assistant. During a meeting, Robertson shared his doubts regarding the existence of gravitational waves, prompting a reevaluation of the Rosen/Einstein paper, which revealed certain errors. Infeld later conveyed his observations to Einstein. In his autobiography, Infeld noted that Einstein recognized and rectified the errors in his scientific paper, while Nathan Rosen maintained his belief that gravitational waves were merely a mathematical construct devoid of genuine physical significance.
The challenge surrounding gravitational waves, as Einstein realized from the outset of deducing their existence, lies in their incredibly minute impact on matter. This is due to the exceedingly small value of the gravitational constant, rendering the prospect of experimental observation seemingly impossible. Furthermore, not all waves are equal; their characteristics are contingent upon the phenomenon that generates them and the influence they exert on matter during propagation. An analogy can be drawn to water waves, where the size of the waves corresponds to the size of the stone thrown into a calm lake. However, it is crucial to acknowledge that this analogy provides a simplistic depiction of reality. Gravitational waves diverge significantly from familiar types of waves, such as sound or electromagnetic waves. Consequently, this distinction forms the focal point of the ensuing scientific discourse within the community.
Chapel Hill Conference
By the mid-twentieth century, gravitational waves had been established as a scientific reality. However, a key question remained unanswered: did these waves possess the capacity to carry energy? With the significant advancements in the theory of relativity and the consequent emergence of new concepts regarding the structure of space-time and the expanding universe, alongside the progress in quantum mechanics and the prospects of unifying it with gravity, the Chapel Hill Conference was convened in 1957. This conference aimed to explore various scientific topics, with a specific focus on gravity and its waves. Over the course of six days, around 40 speakers from eleven countries congregated at the University of North Carolina at Chapel Hill, primarily comprising the new generation of physicists. Among the attendees was the young physicist Richard Feynman, renowned for his adeptness in conceptual and formal experimentation, much like Einstein and Schrödinger. During the conference, Feynman presented an argument known as the “sticky bead argument.” This argument can be succinctly summarized as follows: Imagine the presence of two rings that are not fixed on a rod (as depicted in the upper part of the figure). If gravitational waves exist, they would generate tidal forces, leading to longitudinal pressure on the rod. Since the bead rings are free to move, they would respond to these forces by sliding towards the ends of the rod, away from the center (as shown in the lower part of the figure). This movement would generate friction, resulting in the heating of both the beads and the rod. This thermal effect indicates that energy has been transferred to the rod by the gravitational wave, providing evidence that gravitational waves do carry energy.
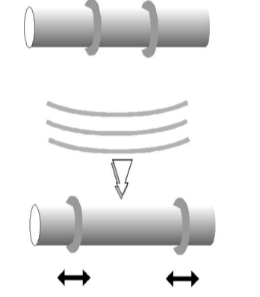
Discussions at the Chapel Hill conference on the effects of gravitational waves persuaded many attendees, including the initially skeptical Hermann Bondi. While theoretical physicists later debated the nature of these waves, an applied physicist took things a step further. Joseph Weber, captivated by the idea, decided to build a device to detect them. His approach focused on measuring vibrations in a mechanical system. He designed a metal cylinder as a kind of antenna, hoping to pick up the resonant vibrations caused by gravitational waves. Similar to how a human ear detects sound waves from a struck bell, Weber envisioned his metal cylinder acting as a gravitational wave “ear.” Over ten years, Weber and his team refined their device several times. However, reactions to his findings were mixed. While some saw promise, others pointed out that gravitational waves, unlike sound waves which compress and disturb air molecules, actually expand and compress spacetime itself.
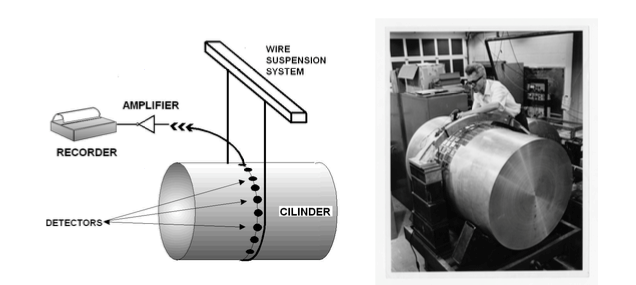
LIGO Project
While the specific inventor of utilizing light properties to detect gravitational waves remains unknown, the concept naturally emerged for many physicists following advancements in optics and laser technology. However, a major hurdle lies in constructing an observatory capable of surpassing all external noise sources. This includes disturbances ranging from everyday phenomena like passing vehicles and seismic tremors to subtle ground vibrations, material interactions, and even the inherent fluctuations of atomic particles. The immense weakness of gravitational waves, with their impact on matter amounting to barely a thousandth of the nucleus’ diameter, amplifies the challenge. Consequently, even the inherent “noise” of the quantum realm can disrupt the measurement process.
In a fortuitous echo of the collaboration that led Robertson and Infeld to review Einstein’s paper and confirm the existence of gravitational waves, destiny intervened once again. In the summer of 1975, Reiner Weiss found himself sharing a ride from the airport with theoretical physicist Kip Thorne, as they both made their way to a NASA meeting on the practical applications of space research in the fields of relativity and cosmology. During their journey, Weiss presented his ambitious proposal: to construct a “space laser interferometer” that could directly detect gravitational waves. One of the PhD students present at the meeting later described the idea as audacious, yet acknowledged that given the technological limitations of 2035, it may also be slightly naive.
Washington was hot and full of tourists, and Thorne didn’t have a hotel reservation so he shared a room with Weiss and they discussed the search for gravitational waves all night. Weiss had been fascinated by the idea of searching for gravitational waves since the early 1970s, and Thorne had written a book called Gravitation in which he expressed his doubts about the possibility of detecting them. Thorne was not convinced at first, but changed his mind after hearing about Weiss’s simple and genius idea, which is the same idea that LIGO is based on.
LIGO, short for Laser Interferometer Gravitational-Wave Observatory, operates by splitting a laser beam into two. One half travels straight, while the other reflects off a mirror perpendicularly. Both beams then return and recombine. If they perfectly overlap, no gravitational waves have passed through. However, the slightest mismatch in their wavelengths indicates the passage of a gravitational wave, which stretches and compresses spacetime, altering the beams’ alignment and disrupting their interference.
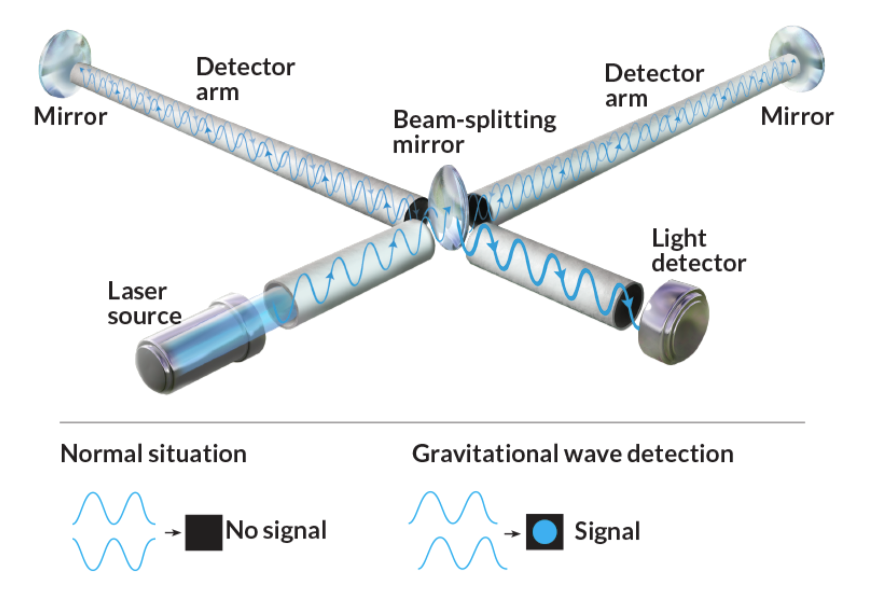
The project was sponsored by the California Institute of Technology (Caltech) and the Massachusetts Institute of Technology (MIT) with funding from the National Science Foundation (NSF). However, the project faced significant delays and funding issues during its early stages in the 1980s. These delays were primarily caused by technical discussions and insufficient funding. It wasn’t until the National Science Foundation took full control and resolved the project’s establishment in 1994 that progress was made. Rochus E. Vogt, the former director of the NSF, was removed from his position due to the substantial delays under his leadership. Physicist Barry Clark Barish was appointed as his replacement. Barish, an experimentalist in high energy physics, had significant experience in managing large physics projects. Under Barish’s administrative leadership, the project received improved financial support. By the end of 1994 and the beginning of 1995, two observatories were constructed approximately 1,900 km apart. One observatory was built in Hanford, Washington State, and the other in Livingston, Louisiana. Both observatories were completed by the end of 1997. Barish’s management approach included the establishment of two separate laboratories. One laboratory focused on the technical and engineering aspects of the observatories, while the other was responsible for scientific research and headed by Weiss. Barish’s philosophy aimed to continually enhance the technical components of the observatory, such as improving the quality of mirrors, optimizing lens focusing, and exploring new materials that were more cost-effective and had greater capacity. Additionally, Barish sought to enable other scientific institutions to benefit from the research conducted at the observatories while also providing financial support for the project.
The project commenced its operations in 2002, but no gravitational waves were detected until 2010. Consequently, the project underwent a five-year closure in 2010 to undergo extensive upgrades. The magnitude of the efforts undertaken by a substantial team of researchers, engineers, and programmers working towards a unified objective cannot be adequately described. Their collective goal was to enhance the sensitivity and precision of the devices, with the ultimate aim of reducing terrestrial and material noise for the sake of a cosmic message.
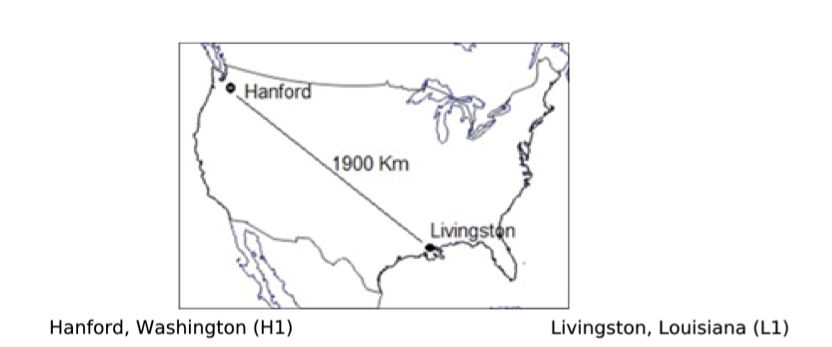
There are two LIGO observatories located in the United States: one in Hanford, Washington, and another in Livingston, Louisiana.
Tango
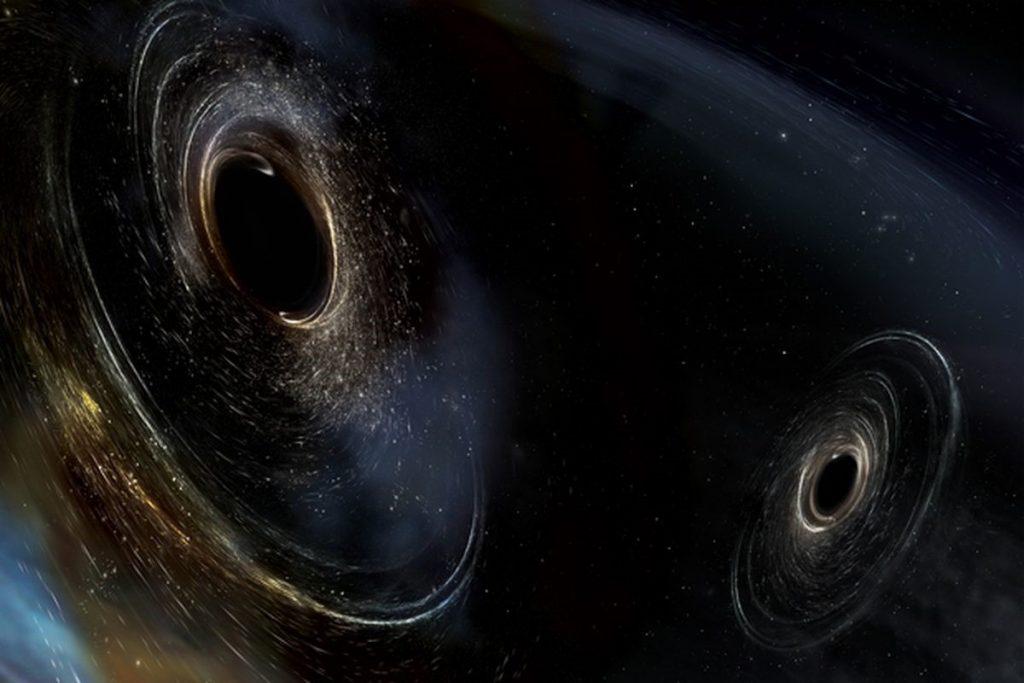
In a remote corner of the universe, situated approximately 1.3 billion light-years away, where life on Earth was yet to exist, an extraordinary cosmic event unfolded unnoticed. Two black holes, one surpassing 36 solar masses and the other exceeding 29 solar masses, engaged in a mesmerizing celestial ballet. With the elegance of tango dancers, they spiraled around each other at immense velocities until eventually merging into a single black hole with a mass greater than 62 solar masses. The remnants of their masses coalesced into concentrated three solar masses, forming a remarkable spectacle. This cosmic spectacle sent forth gravitational waves, which traversed the fabric of space-time, passing through us and everything in the universe, as we intersected with these gravitational waves on 25th September 2015. Their impact extended to our very atoms and those of every element in existence. The waves caused infinitesimal expansions and compressions, represented by an incredibly minuscule value (a decimal point followed by twenty zeros and then a one). This minute vibration induced by gravitational waves serves as the fundamental principle behind the concept of LIGO. LIGO, founded on a daring, ingenious, and profoundly imaginative idea, capitalizes on the remarkable property of light called “interference.” By measuring the disturbances in a laser beam resulting from turbulence, the LIGO project embarked on a journey that defied conventional norms. It can be described as an audacious endeavor, driven by a blend of madness, brilliance, and boundless creativity.
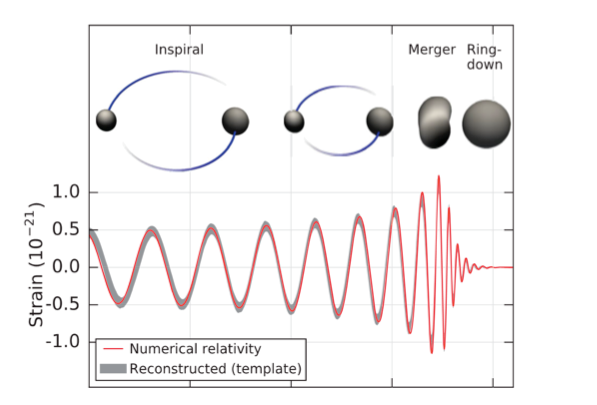
The signal generated by the rotation and subsequent merger of the two black holes, culminating in the formation of a final black hole.
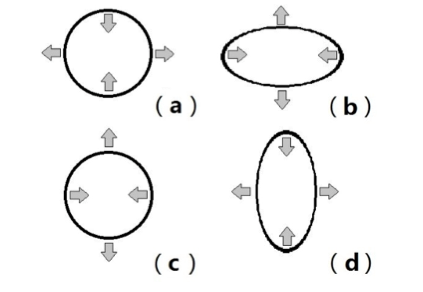
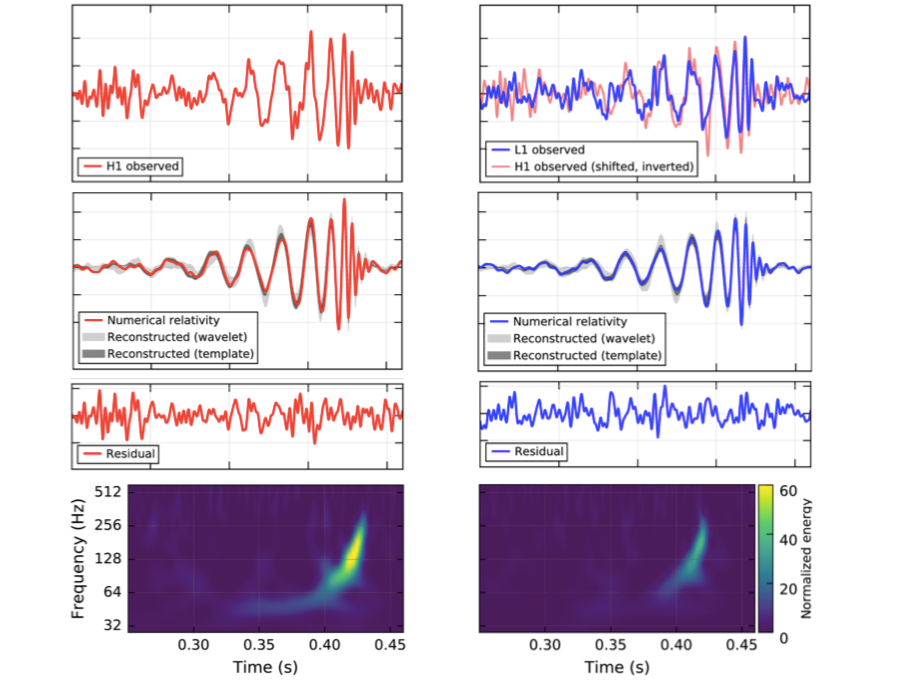
“It was too good to be true”
These words were spoken by Kip Thorne, who conveyed his profound sense of joy upon hearing the detection of gravitational waves and witnessing their fleeting signal, lasting less than a second. Both the Hanford and Louisiana observatories captured the same wave, but it took the scientific team a considerable amount of time to analyze the data. At the LIGO Observatory, there exists a covert team specialized in generating simulated signals or pulses to test the laboratory personnel. Following confirmation from the administration to refrain from sending test signals, the observatory refrained from making any announcements and maintained complete secrecy. This approach aimed to ascertain whether additional gravitational waves were being detected. The second wave was indeed observed in December 2015. On February 11, 2016, LIGO finally stepped forward to announce this remarkable achievement. The news was met with immense joy within the scientific community and received widespread media coverage. In recognition of their contributions, Reiner Weiss, who conceived the idea of observing gravitational waves using laser interferometry, Barry Barish, the director of the LIGO project, and theoretical physicist Kip Thorne, who made significant scientific advancements in gravitational wave calculations and technical contributions to the observatory, were jointly awarded the Nobel Prize in Physics in 2017.
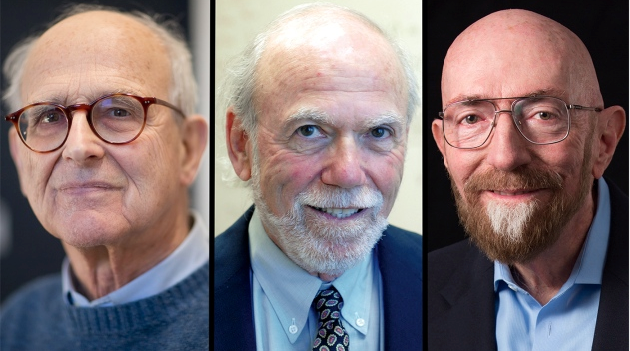
The Future of Gravitational Waves
Yes, of course, I have pondered the idea of constructing an observatory like LIGO, but not on Earth, rather in space. This very idea was proposed by Rainer Weiss in 1975, and he is determined to bring it to fruition. The European Space Agency (ESA) has taken up the mantle of this project, aptly named LISA, which stands for Laser Interferometer Space Antenna. The concept entails deploying three satellites in the shape of an equilateral triangle. Each satellite would be equipped with mirrors and light detectors, facilitating the transmission and reception of laser beams simultaneously. These three satellites would be arranged in orbits that align with the Earth’s orbit. The motivation behind choosing space as the observation site stems from the desire to eliminate all terrestrial noise caused by natural phenomena such as earthquakes, volcanoes, and construction-related vibrations. Furthermore, capturing the gravitational waves originating from physically large events necessitates long and straight detector arms, a feat that cannot be achieved on Earth. In the LISA observatory, the estimated length of the arms is approximately 2.5 million kilometers. The project is anticipated to reach completion by 2034, marking a significant milestone in our quest to explore the cosmos.
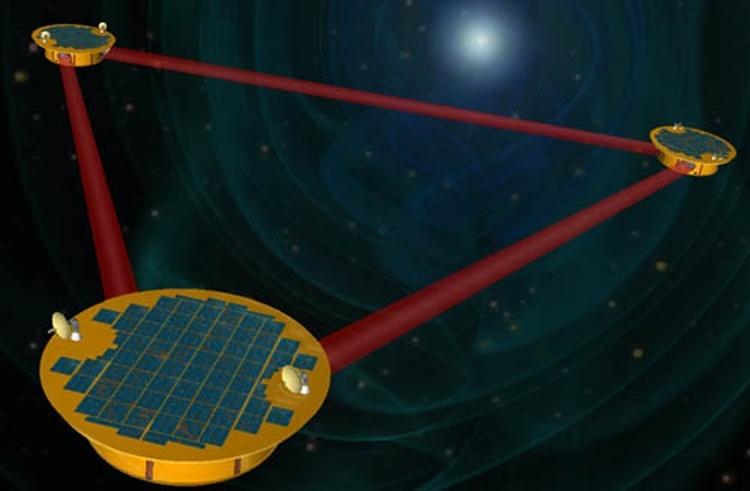
Messengers of the Universe
Perhaps the question that has frequently been asked, and continues to be asked, is: What will gravitational waves contribute to humanity?
First, observing gravitational waves represents the latest experimental evidence supporting one of the predictions of general relativity. In the scientific community, general relativity now stands on solid and well-established foundations of scientific reliability, with the confirmation of the following five predictions:
- The deflection of light and the distortion of space-time, as demonstrated during the 1919 solar eclipse.
- The expansion of the universe, confirmed in 1929.
- The existence of black holes, proven in 1970.
- The observation of Einstein’s ring (gravitational lens) in 1998.
- The detection of gravitational waves in 2015.
Second, four years after the first observation of gravitational waves, a total of 23 gravitational wave events have been confirmed. Among these, 20 resulted from the merger of black holes, two from the merger of neutron stars, and one from the interaction between a black hole and a neutron star. This last phenomenon represents a completely new discovery in the field of cosmic physics. Scientist Mavalfla from the Massachusetts Institute of Technology expressed her astonishment at the significant advancements in observatory instruments during the years 2018-2019, stating that “the field of gravitational waves has exploded.” It is anticipated that by the year 2023, gravitational waves will be observed on an hourly basis. This progression will lead theoretical physics and cosmology towards a path of enhanced observation, measurement, and numerical relativity.
Third, the advancements in quantum computing have greatly facilitated the study and analysis of complex equations pertaining to the positions, masses, and velocities of black holes. These advancements have enabled researchers to apply these equations to the principles of general relativity. This mutually beneficial relationship between gravitational waves, precise observations, and technological applications has the potential to drive further progress in our understanding of the universe.
Fourth, one of the most significant puzzles in cosmology revolves around the formation of supermassive black holes at the centers of galaxies. Solving this puzzle would also provide insights into the formation of galaxies themselves. Detecting a gravitational wave that has traveled 1.3 billion light-years indicates that we are approaching the ability to observe the most crucial wave in existence—the gravitational wave that occurred immediately after the Big Bang. This realization brings us closer to unraveling the mysteries of the universe.
Fifth, this remarkable achievement for humanity alleviates doubts regarding the allocation of funds to scientific institutions. It fosters increased confidence in financing and supporting these institutions. The LIGO management, for example, has made significant advancements by enhancing the sensitivity of their devices, increasing their capabilities from two to four or even six times. Furthermore, countries have engaged in healthy competition to construct their own gravitational wave observatories. Presently, there are five new observatories situated in different parts of the world, including France, Italy, the Netherlands, Japan, and India. The existence of multiple observatories in diverse locations offers greater precision in identifying the sources of gravitational waves and determining their originating masses.
Sixth, gravitational waves serve as cosmic messengers, enabling us to decipher numerous astronomical phenomena such as black holes, white dwarfs, and supernovae. They also serve as a testing ground for theories in cosmology, including dark energy, dark matter, and quantum gravity. Gravitational waves shed light on the many enigmatic aspects of our universe that remain clouded in our limited understanding. In fact, it is plausible that gravitational waves may even lead us to the discovery of previously unknown phenomena, analogous to the tale of the Russian sailor Anatoliy’s ship.
References:
- A Brief history of gravitational waves. Jorge Cota; Salvador Galindo;Georg Smoot. September 2016.
- The Gravitational-Wave “Revelution” is underway. September 2019, https://www.scientificamerican.com/article/the-gravitational-wave-revolution-is-underway/
- https://www.ligo.caltech.edu/page/press-release-gw170104
- Gravitational Waves: A New Era of Astronomy Begins; 22/06/2016, https://www.youtube.com/watch?v=xj6vV3T4ok8
- The Future of Gravitational Wave Astronomy; 9/08/2019, https://www.youtube.com/watch?v=jKrOy4mC4wg
- The Future of Gravitational Wave Astronomy; 25/06/2017, https://www.youtube.com/watch?v=eEaaGTV0V2Y
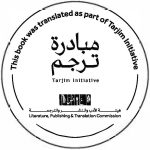
T1618